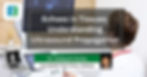
In the Article - Discover the intricacies of ultrasound propagation in tissues with our latest blog post, 'Tissues: Understanding Ultrasound Propagation'. Learn about reflection, refraction, scattering, absorption, and more!
Introduction - The ultrasound waves interact with the tissues in various ways, such as reflection, refraction, scattering, absorption, and attenuation. These interactions depend on the physical properties of the tissues, such as density, elasticity, impedance, and acoustic velocity. Moreover, the tissues are not homogeneous and isotropic but rather have complex and dynamic structures that vary with time and space. Therefore, understanding how ultrasound waves propagate in the tissues is essential for improving the quality and accuracy of ultrasound imaging.
Ultrasound Propagation – Ultrasound propagation is the process of how ultrasound waves travel through different media, such as air, water, or tissues. Ultrasound propagation depends on the physical properties of the medium, such as density, elasticity, impedance, and acoustic velocity.
The propagation speed of an ultrasound is how fast an ultrasound wave travels with in the tissues.
The speed of sound in a medium depends on two factors: how easily it can be deformed (stiffness) and how much mass it has per unit volume (density). Stiffness and speed are directly proportional, meaning that the stiffer the medium, the faster the sound. Density and speed are inversely proportional, meaning that the denser the medium, the slower the sound. However, stiffness usually has a stronger effect than density on the speed of sound. For example, bone is very stiff and very dense, but sound travels faster in bone than in any other tissue in the human body. The average speed of sound in the soft tissues of the human body is 1540 meters per second, which is the value used by ultrasound machines. However, this value is not accurate for all tissues and can cause artifacts in the ultrasound images when the sound encounters tissues at different speeds.
At normal incidence, a wave encounters a surface perpendicularly, leading to either reflection or transmission of the sound through the medium. This scenario is characterized by the wave's alignment with the interface, defining it as an instance of normal incidence.
Examples of propagation velocities in different tissues
Air: 330 m/sec.
Fat: 1450 m/sec.
Water: 1480 m/sec.
Liver: 1550 m/sec.
Kidney: 1560 m/sec.
Blood: 1570 m/sec.
Muscle: 1580 m/sec.
Bone: 4080 m/sec.
The Dynamics of Ultrasound Waves: Intensity, Power, and Amplitude
Intensity refers to the amount of energy a sound wave carries per unit of time and per unit of area.
Amplitude is the measure of the maximum displacement of particles in a medium from their rest position when the sound wave passes through.
The relationship between intensity and amplitude in sound waves is direct but non-linear.
In general, the intensity of a sound wave is proportional to the square of its amplitude. This means that if you double the amplitude of a sound wave, the intensity increases by a factor of four.
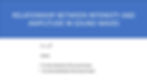
This relationship highlights how changes in amplitude significantly impact the energy and strength of a sound wave. To prevent possible harm to the tissues, we control the intensity, power, and amplitude of the ultrasound waves.
Understanding Attenuation of Ultrasound Waves -
Attenuation of ultrasound waves refers to the gradual decrease in the intensity or amplitude of the waves as they travel through a medium. This phenomenon occurs due to the absorption, scattering, and dispersion of the ultrasound energy by the medium through which the waves propagate. In medical ultrasound imaging, understanding and compensating for attenuation are crucial for obtaining clear and accurate images.
A sound wave characterized by high frequency and a shorter wavelength experiences rapid attenuation, resulting in a swift loss of intensity. Conversely, a sound wave featuring a low frequency and a longer wavelength exhibits a comparatively lower rate of attenuation, leading to a more gradual decline in intensity over distance.
Imagine being at a music concert: as you move farther away, the song's clarity might fade, but the bass notes, marked by their low frequency, persistently reach your ears. This happens because low-frequency sounds, possessing longer wavelengths, undergo a slower rate of attenuation, enabling them to travel more effectively across greater distances.
Let's explore various forms of attenuation in ultrasound.
ABSORPTION: As ultrasound waves traverse through the body, tissues absorb a significant amount of their energy, often converting it into thermal energy or heat. Absorption is a primary contributor to the loss of energy in a sound wave.
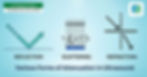
REFLECTION: The foundation of ultrasound imaging lies in reflection. The echo or reflection of an emitted pulse is essential for generating an ultrasound image. Notably, it's crucial to understand that not all sound waves reflect directly back to the transducer; many reflect at angles away from it.
SCATTERING: In soft tissue, ultrasound waves scatter in multiple directions when encountering objects smaller than the wavelength or irregular tissue surfaces. Visualize dropping a pebble into a calm pond, where the resulting ripples mirror the behavior of sound waves undergoing diffuse scattering. Scatter disperses in various directions, including backscatter, directed back toward the transducer.
REFRACTION: Refraction involves a change in the direction of a wave due to a shift in its transmission medium. This occurs when a sound wave transitions from one speed of sound transmission to another, especially when the angle of incidence is not 90 degrees. Similar to light experiencing refraction in media of varying optic densities, sound waves undergo changes in direction during transmission.
Acoustic impedance - Acoustic impedance is a property that characterizes how sound waves propagate through a medium. It is the product of the density of the medium and the speed of sound in that medium. Mathematically, acoustic impedance (Z) is represented as:
Z=ρ⋅c
where:
Z is the acoustic impedance,
ρ is the density of the medium, and
c is the speed of sound in the medium.
Acoustic impedance is a crucial factor in understanding the behavior of sound waves at interfaces between different materials. When sound waves encounter a boundary between two media with different acoustic impedances, some of the wave energy is reflected, and some is transmitted. In medical ultrasound, the impact of acoustic impedance is particularly noticeable at the junctions between diverse tissue types. The transition of sound waves from one medium to another is influenced by the discrepancy in impedance between these tissues. This phenomenon is termed "Acoustic Impedance Mismatch." The extent of this mismatch directly correlates with the magnitude of the reflected echo back to the transducer. Essentially, a greater acoustic impedance mismatch results in a more pronounced echo being reflected.
"specular Reflection" and "Non-Specular Reflection" - In ultrasound imaging, the terms "specular reflection" and "non-specular reflection" refer to two different types of reflections that occur when ultrasound waves encounter boundaries between different tissues or structures.
Specular Reflection: Definition: Specular reflection occurs when ultrasound waves encounter a smooth, flat surface or boundary between tissues. In this case, the waves reflect off the surface at predictable angles.
Characteristics: The reflection is organized and follows the law of reflection, similar to how light reflects off a mirror. Specular reflection results in clear and distinct echoes that contribute to the formation of well-defined images.
Non-Specular Reflection (or Diffuse Reflection): Definition: Non-specular reflection, or diffuse reflection, occurs when ultrasound waves encounter irregular surfaces or boundaries with uneven structures.
Characteristics: Unlike specular reflection, the reflection angles in non-specular reflection are not predictable. The waves scatter in various directions due to the uneven nature of the surface. This type of reflection contributes to the formation of scattered echoes that may not be as organized or distinct as those in specular reflection.
An instance of a non-specular reflector is found in red blood cells. Due to their size being considerably smaller than the wavelengths of ultrasound, red blood cells scatter sound waves. This form of scattering observed when the reflector's dimensions are much smaller than the ultrasound beam's wavelength, is termed Rayleigh scattering.
About the Author -
Dr. Debjyoti Dutta, an esteemed pain physician and author, is affiliated with Samobathi Pain Clinic and Fortis Hospital in Kolkata. Holding the position of registrar at the Indian Academy of Pain Medicine, he specializes in musculoskeletal ultrasound and interventional pain management. Dr. Dutta, recognized internationally, has authored significant works such as "Musculoskeletal Ultrasound in Pain Medicine" and "Clinical Methods in Pain Medicine," providing in-depth perspectives on the field of pain management. Dr Debjyoti Dutta is one of the faculties of Asian Pain Academy Courses which provides the best pain management fellowship training in Kolkata, India.